[ad_1]
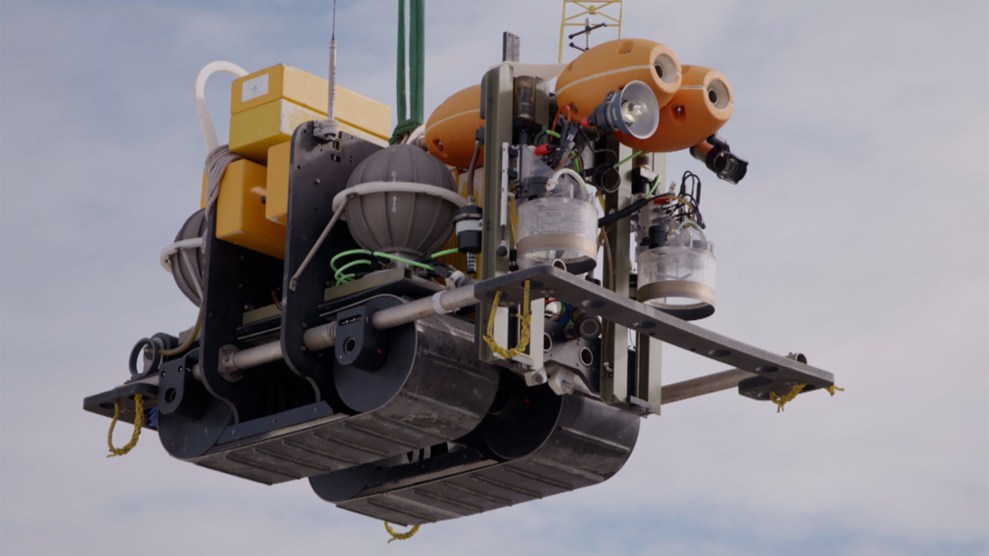
The Benthic Rover II is a cleverly designed device that can roam the seafloor for up to a decade at a stretch.Madison Pobis, Copyright 2021 MBARI
This story was originally published in WIRED It is reproduced here as part the Climate Desk collaboration.
The Benthic Rover II isThe size of a compact car, although it rocks fat treads, making it more like a scientific tank. This, along with two flotation devices that look like googly eyes, gives it a WALL-E-like vibe. BR-II, however, does not explore a trash-strewn landscape. Instead, it roams the Pacific seafloor at 13,000 feet. The robot’s mission: To prowl the squishy terrain in search of clues about how the deep ocean processes carbon.
The mission begins with a wild ride around the coast of Southern California, 180 miles. Scientists at the Monterey Bay Aquarium Research Institute lower BR-IIInto the water and then … drop it. Completely untethered, the robot free-falls for two and a half hours, landing on the abyssal plains—great stretches of what you might generously call muck. “It’s mushy and dusty at the same time,” says MBARI electrical engineer Alana Sherman, coauthor on a new paper in Science Robotics describing findings from the robot’s adventures. “Which is part of the reason it’s a tracked vehicle, and it has these really wide treads.” That extra surface area distributes the robot’s weight so it doesn’t sink into the sand.
The deep sea is the ideal place to torture a robot. At these depths the water is cold, salty (and therefore corrosive), and highly pressurized; there’s a whole lot of liquid pushing down on the robot.
Like the Mars roversThis robot must be self-sufficient. In fact, in some ways it’s even More difficult to keep tabs on a rover 13,000 deep than it is a rover on another planet. Radio waves travel well in space, it’s just that they take up to 20 minutes each way to make the trip between Earth and Mars—and good luck remotely piloting a rover in real time with that kind of delay. Radio waves are the only way to communicate. Hate water. Instead, BR-II uses acoustic signal to talk to another robot. MBARI scientists release a floating glider from shore four times a calendar year. The glider, essentially a very expensive surfboard, travels to the rover’s approximate location, pings it, collects status updates and sample data, and fires that information to a satellite for the researchers to access.
Since MBARI scientists can’t just sit in their labs and pilot the rover, it’s on its own. Its instructions are easy. It is placed on the seafloor and lowers the oxygen sensors into the muck. This allows the robot to measure the biological activity of sediment by lowering two oxygen sensors into the muck. Microbes consume oxygen and expel carbon dioxide. Fluorescence camera technology is also used to make organic matter glow. This gives the robot an idea of how much detritus from surface waters, known as “marine snow,” is making its way down to the seafloor.
The rover will remain in the same place for 48 hours and then move forward 33 feet. That’s all. “It would not know if it drove off a cliff—all it knows is I’m supposed to drive forward 10 meters,” says Sherman. “But luckily, there are no cliffs around, so we take advantage of the simplicity of the environment to keep the robot more simple.”
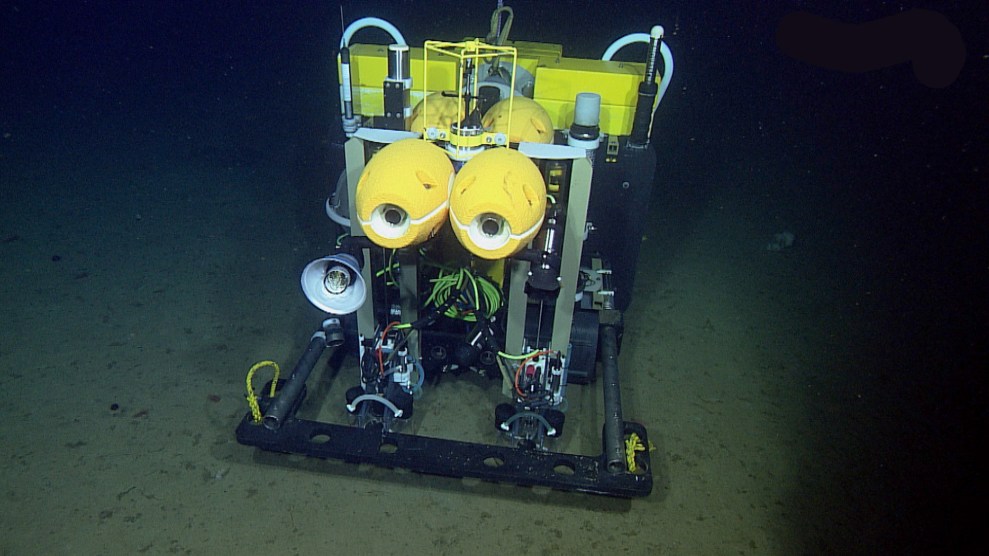
The two oxygen samplers can be seen below the eyeball floats. Copyright 2016.
MBARI
Still, there’s a problem: The oversized treads make a mess of the seafloor. “Even though it is moving very slowly, it doesn’t take much to create this huge dust storm,” says Sherman. “We always want to be driving into the current, so that it can push the sediment that is disturbed behind us.” So before the rover moves, it uses a sensor to get an idea of the current direction of the … er, current, then heads straight for it.
This is how the benthic rover goes for a whole year unsupervised: park, take measurements and move 33 feet. Repeat. The scientists then take the boat out to give it a new battery.
At the back of the robot are two titanium spheres—each somewhere between the size of a yoga ball and a beach ball—filled with batteries that power a year of continuous operation. When it’s time to resupply power, the scientists retrieve BR-11 by sending it a signal that releases a 250-pound weight attached to the robot’s belly. Those flotation devices that look almost like eyes start to work once the weight has been dropped. They are actually “syntactic” foam: Instead of being mushy, porous plastic filled with air, they are actually made of hard material and filled with small glass spheres, each containing air. The robot can propel itself to the surface even under pressures that would make normal foam collapse in on themselves.
The scientists haul the rover up on board their boat, download BRI-II’s data, swap out its batteries, and check it for problems. If everything’s good, they release it to spend another year roaming the abyssal plains. The last time the scientists went out, though, they discovered that one of BR-II’s motors had failed, so they had to bring it ashore for repairs. They ended seven years of uninterrupted operation. This was the end of an amazing story, which they recount in their current paper.
MBARI scientists have gained unprecedented insight from this long observation period into the workings of the deep. This includes both large stretches of seafloor and long timescales. That will be critical to understanding our planet’s carbon cycle. At the ocean’s surface, a galaxy of algae known as phytoplankton sequesters carbon, the way plants do on land. The algae are then eaten by tiny animals called zooplankton. The carbon-rich pellets are then absorbed into the water column and become marine snow. Some of the waste gets eaten, either along the way or by bottom-dwelling creatures, but the rest becomes sequestered in the sediment, locking the carbon far away from Earth’s atmosphere.
But the amount of carbon that gets trapped can vary greatly from one ocean to the other and from one season or another. In general, researchers just don’t have a good handle on the biological and chemical processes going on down there. “The rover helps us understand how much of that carbon might actually make its way into the sediments in the deep sea,” says MBARI marine biologist Crissy Huffard, who coauthored the new paper. “It’s our only view into how much carbon might actually get stored into the sediments, versus how much actually is consumed and probably contributing to acidification in the deep sea.” (When carbon dioxide dissolves in seawater, it forms carbonic acid.)
Here’s a tricky example of one of those seafloor carbon mysteries. California’s land is heating faster than the surrounding ocean, which intensifies the seasonal winds. That could be driving more upwelling—wind pushes the surface water away, and water from below rushes up to fill the void. This would result in more nutrients that could be used to feed phytoplankton. These organisms then bloom in surface waters before dying and becoming marine snow. Between the years 2015 and 2020, for instance, BR-II’s fluorescence camera detected a massive increase in the amount of phytoplankton reaching the seafloor in big pulses. It also detected a decrease of oxygen, which meant that the seafloor microbes were busy processing the organic material.
Huffard is left with some questions. “Just in general, the area’s becoming a lot more erratic in its food supply—it can be years’ worth of food coming down in a few weeks. So how is that changing the whole ecosystem?” she asks. “The response by the animal community is almost instant. They start consuming it right away, there’s no big lag. The microbes are just primed and ready to go.”

A rattail fish captured on BR-II’s camera. Copyright 2021
MBARI
What does this all mean for the carbon cycle Theoretically, the more organic material that’s raining down, the more that’s getting sequestered away from the atmosphere. However, organisms living on the seafloor are also consuming oxygen and spitting carbon dioxide, which could be acidifying deeper water. Because the ocean is constantly turning, some of this carbon may make its way back to the surface waters and into atmosphere. “We’re showing that more and more carbon than would have otherwise been predicted is making its way to the deep sea,” says Huffard. “The rover adds the dimension to tell us that most of that carbon is actually getting eaten once it’s down there, not being stored in the sediment.”
These extra-large pulses are marine snow, but is this a permanent feature in the deep waters of California? Scientists can now gather long-term data using the benthic Rover to help them find answers. “The deep sea is largely understudied and under-appreciated, despite the fact that it is critical to keeping the planet healthy and combating climate change,” says Lisa Levin, who studies the seafloor at the Scripps Institution of Oceanography but wasn’t involved in this work. “An army of such devices could help us better understand biogeochemical changes—critical to improving climate models, ecosystem models, fisheries models, and more.” Rovers might also help scientists study the effects of deep-sea mining operations.
For now, Huffard and Sherman will keep BR-II rolling off the coast of California—the first of hopefully many such autonomous bottom-dwelling robots that could roam the depths of the world’s oceans. They say they’ve been approached by other scientists interested in the system, but so far BR-II is more or less one-of-a-kind, both because it’s expensive and requires a lot of engineering know-how to operate. German researchers have created a similar oxygen-sampling rover called TramperThe Arctic has been home to the, which has been exploring the Arctic since 2016.
“It’s almost like if you were an astronomer and if you have the best telescope in the world, but it could only look at one star,” Huffard says. “If you had more telescopes out there looking at more stars, you’d be able to see a much more complete picture of the sky.”
“I think we would both love it if more people wanted to build more rovers,” she adds.
Sherman laughs. “As long as they don’t call us to fix them.”